Journal Search Engine
ISSN : 2234-1099(Online)
DOI : https://doi.org/10.5000/EESK.2013.17.4.181
Analytical Studies on Seismic Performance of Multi-Story Coupled Piping System in a Low-Rise Building
Abstract
- (4)13-03.pdf1.96MB
1. Introduction
Over the past few decades, the seismic design for the structural systems has been significantly improved. According to Parulekar (2009), previous seismic safety criteria related to structures of nuclear power plants and hospital facilities were designed less rigorous than the present design codes. Therefore, in order to meet the current design criteria, some structures needed to retrofit or strengthen by various devices such as fiber wrapping, steel and concrete jacking, and passive/active dampers (Parulekar, 2009). However, damages from nonstructural components such as mechanical/electrical equipment, ceiling, and piping system were still observed during earthquakes.
During 1994 Northridge earthquake, approximately 85% of the total damage cost, which was estimated about $7.4 billion, was attributed to non-structural systems (Kircher, 2003). For example, the Olive View Hospital had to be shut down shortly after the earthquake due to water damage caused by failure of sprinkler systems (Ayer & Phillips, 1998). Similarly, during the 1971 San Fernando earthquake, 4 out of 11 medical facilities in the area experienced significant economic losses due to the damages on the nonstructural components (Wasilewski, 1998). Damage to components such as fire protection piping system, Heating, Ventilating, and Air Conditioning (HVAC), and water piping systems have resulted in direct economic loss and caused causalities such as injuries or loss of life in many seismic events.
In recent years, many researchers have recognized a need to address the challenges during the designing stages such that the nonstructural components remain operational or functional during and after an earthquake. Antaki and Guzy (1998) conducted static and dynamic tests of the fire protection piping systems designed in accordance with National Fire Protection Association (NFPA-13). The objective of their tests was to identify the stiffness, failure modes, and limit states for leakage of threaded pipe joints and grooved coupling systems that are commonly used in the piping systems. Maragakis et al. (2003) identified the capacity characteristics of a welded hospital piping system with and without bracings as well as the system’s weak points. Lee (2008) characterized the analytical modeling of fire protection piping system using Finite Element (FE) platform, ANSYS. Seismic behavior of the piping system based on design spectrum was evaluated in this study. ATC-58 has highlighted the need for a performancebased design approach in nonstructural systems to mitigate seismic risk and achieve reliable designs. Therefore, due to the high cost associated with the repair and reinstallation of the piping systems, the seismic performance evaluation and design of nonstructural systems has received significant attention in recent years.
The primary objective of this study was to develop a framework for evaluating seismic performance of multi-story fire protection piping systems and water distribution piping systems using multiple time history analyses. In order to identify and understand the tuning effect between a 5-story building system and its piping system, analyses of multiple time histories by OpenSees platform were conducted for a single-story piping on top floor and a multi-story piping system in the building system. Due to the complexity of the piping system, the multiple linear time history analyses were conducted in this study.
2. Numerical Modeling of a 5-Story Building System
Based on the building design from Wood et al (2009), a 5-story steel frame building system was selected for this study. The building system designed according to a strong column and weak beam frame design philosophy has been modeled in Open System for Earthquake Engineering Simulation (OpenSees) based on structural analysis of Finite Element (FE) method by Tck/Tk interpreter extension (Mazzoni et al., 2006). Figure 1 showed a schematic design of the 5-story steel frame building system. In order to simplify the analysis, only a single bay system was adopted and the span of single bay was designed as width 6.0 (m) by height 5.5 (m) for the first floor and width 6.0 (m) by height 4.0 (m) for other levels, respectively. In addition, the soil and structure interaction effects were not considered in this study.
Fig. 1. Steel Frame Building Model Description
The numerical discretization of the steel frame building was modeled with an elastic uniaxial material. The elastic Beam-Column element in OpenSees was designed to characterize the response from the beam and column section under different earthquake ground motions. Furthermore, lumped mass and equivalent loads were used and the damping ratio using mass and stiffness proportional Rayleigh damping in all modes distribution to the response in steel building model was taken as 2%.
The fundamental and second frequencies conducted from an eigenvalue analysis for the building system was 2.5891 and 9.0643 (Hz), respectively. Also, the mass participation of the fundamental and second mode was 78.37 % and 12.72 %, respectively. As such, the mass participation over 90 percent was dominated by the first two modes in the building system.
3. Numerical Modeling of a Single-Story and Multi-Story Piping Systems
In order to identify seismic performance of piping system installed in the steel building system, the fire protection piping system in Figure 2 was selected. Typically, for multiple time history analyses, the piping system in the rectangular area was selected as seismically critical zone. Briefly, a single-story fire protection piping system was located on top floor of the building, and it incorporated with a horizontal main piping system with 14 sub-branch piping system (Figure 3). As can be seen in Figure 4, the multi-story piping system was modeled from ground level to top floor level in a 5-story building. The piping system was incorporated with a vertical main piping system and horizontal main piping systems with the branches of each floor. To represent a pressure vessel at the ground level, lumped mass of 1000kg was included in the multi-story piping system.
Fig. 2. Current Piping System Installed in a Building
Fig. 3. Finite Element Modeling of the Single-Story on the Top Floor by OpenSees
Fig. 4. Finite Element Modeling of the Multi-Story Piping System by OpenSees
Essentially, main piping system was made of 5-inch schedule 40 Black Iron pipe while the branches comprised of pipes with smaller diameter (1-inch) than those of main pipes. This single and multi-story piping system was supported by unbraced single hangers, transverse/longitudinal braced hangers, and anchors.
As described in above building section, the numerical discretization of the piping systems was also modeled with elastic uniaxial material and used lumped mass. The damping ratio using Rayleigh damping method in all modes was also taken as 2%.
Table 1 showed the natural frequencies of the piping systems. The fundamental and second mode frequencies of two different piping systems were almost similar. Also, the frequencies of the first 5-mode in the multi-story piping system were very closely together.
Table 1. The Natural Frequencies of the Piping Systems
4. Selected Ground Motions
In order to evaluate seismic performance of the piping systems in this study, 5 selected earthquakes from PEER-NGA database (PEER, 2009) were considered as ground motion uncertainty associated with their fault mechanism. Table 2 showed the details of the ground motions considered in this study and Figure 5 gave the response spectra with 5% damping ratio for the 5 earthquakes normalized to the same PGA, 1g.
Table 2. Earthquake Ground Motions
Fig. 5. Response Spectra with 5% Damping Ratio
5. Seismic Performance Evaluation of Piping Systems
As can be seen in the configuration of the piping systems, the piping systems were very complicated and the piping system was commonly connected with various joints such as threaded T-joint, grooved T-joint, and elbow connections. Due to the prevalence of significant higher mode effects, the complexity of the failure mechanism was also enhanced. Moreover, the common damage or failure patterns have been identified to exist at more than one location in a piping system. Therefore, the primary goal of this study was to characterize the seismic performance of a single-story and multi-story piping system due to interaction with a low-rise building. In this section, the time history analyses were conducted by OpenSees platform for the piping systems, and the direction of the earthquake was applied to two different horizontal directions. More specifically, in order to determine the critical locations at the connections in the piping system, the multiple linear time history analyses were preformed. The analyses in the piping systems were evaluated as followings:
Step 1: Analyze the building system subject to 5 different earthquakes by linear time histories.
Step 2: Obtain the acceleration and displacement time histories from each floor in the building system.
Step 3: Apply the acceleration time histories on the top floor to the single-story piping system, while simultaneously applying the displacement time histories of each floor to the multi-story piping system from ground level to top floor level using the multiple-support excitation in OpenSees platform.
Step 4: Evaluate the seismic performance of the single and multi-story piping system from two different horizontal directions.
The displacement responses on the top floor of the single-story and multi-story piping system subjected to the seismic ground motions was listed in Table 3. Location 1 connected with elbow system was a critical location of x-direction and location 2 connected with T-joint system was another critical location of z-direction for the piping systems. The maximum displacements between the single-story and multi-story piping system on the top floor were slightly different. However, the maximum displacement from the Taiwan earthquake was significantly increased to the multi-story piping system on the top floor level. Figure 6a and 6b showed the maximum displacements on each floor for the multi-story piping system in each direction of the earthquakes. As shown in these Figures (Figure 6a-6b), the maximum displacements increased linearly with increasing floor level of the multi-story piping system. Taiwan and Northridge earthquake led to the excessively large displacements (194.76 and 126.12 cm) of the multi-story piping system of the top floor at location 1 and 2, respectively. In contrast, the largest force (93.72 KN) of the piping system at location 1 was observed from the Turkey earthquake. Furthermore, the force-displacement relationships on the top floor for the multi-story piping system subjected to 5 different ground motions were shown in Figure 7. Figure 8 illustrated the force-displacement relationships on the each floor for the piping system from the El-Centro earthquake.
Table 3. The Maximum Displacements of the Piping Systems at Each Direction
Fig. 6. Maximum Displacements of the Multi-Story Piping System
Fig. 7. Force-Displacement Relationships from 5-Different Earthquakes on the Top Floor at Location 1
Fig. 8. Force-Displacement Relationships of the Multi-Story Piping System Subjected to El-Centro Earthquake
6. Conclusion
The construction cost of nonstructural systems account for a significant proportion of total cost. Furthermore, damage to the nonstructural systems in critical facilities comprise a significant proportion of the total economic loss incurred in the event of an earthquake. Therefore, it was necessary to understand the seismic performance of the complex piping systems a nonstructural system. This paper focused on the evaluation of the seismic characteristics of the single-story on the top floor and the multi-story piping system in 5-story steel frame building system as decoupled analysis between piping system and building system. The analytical conclusions of this paper were as followings:
1) For consideration of ground motion uncertainties, 5 different earthquakes distributed in different geographical regions were used. The maximum displacements of the piping systems on the top floor between the single-story and the multi-story piping system were close to each other approximately. However, the maximum displacements between two piping systems subjected to Taiwan earthquake was significantly different because the Taiwan earthquake was tuned at low frequency level as shown in the response spectra figure. In addition, the performance was significantly changed due to higher mode effects of piping systems and tuning effect between the multi-story piping system and the building.
2) The maximum displacements and forces on each floor of the multi-story piping system showed linear increment along with interaction with building performance in both horizontal directions. It showed that Current piping system in South Korea was not properly designed in accordance to design force levels. In another word, current piping system on top floor level was designed with same force level as the piping system applied on the ground level. Therefore, it is necessary to strengthen the piping system with seismic sway bracing (i.e. transverse and longitudinal braced hanger systems) based on the floor height of the building.
Further, performance-based earthquake engineering for risk and safety assessment based on probabilistic framework of the piping system must be evaluated and nonlinear performance of the complex piping system such as T-joint, elbow, and supporting systems must be achieved.
Acknowledgement
This work was supported by the National Research Foundation of Korea (NRF) grant funded by the Korean government (MEST) (No. 2012-0008762).
Reference
2.Ayres JM, Phillips RJ. Water Damage in Hospital Resulting from the Northridge Earthquake. ASHRAE Transactions Part 1B. 1998; 104:1286-1296.
3.Korea Meteorological Administration (KMA). 2012 Geological Distribution of Earthquakes [Internet]. 2013.Available from: http://web.kma.go.kr/notify/press/kma_list.jsp?bid=press&mode=view& num=1192542.
4.Kircher CA. It Makes Dollars and Sense to Improve Nonstructural System Performance. Proceedings of Seminar on Seismic Design, Performance, and Retrofit of Nonstructural Components in Critical Facilities. ATC-29-2. Newport Beach, California. c2003.
5.Lee D. A Study on the Seismic Design for Water Extinguishing Piping Systems. Journal of Korea Institute of Fire Science and Engineering. 2008;22(1):10-15.
6.Maragakis E, Itani A, Goodwin E. Seismic Behavior of Welded Hospital Piping Systems. Proceedings of Seminar on Seismic Design, Performance, and Retrofit of Nonstructural Components in Critical Facilities. ATC-29-2. Newport Beach. California. c2003.
7.Mazzoni S and et al. OpenSees Command Language Manual. Open System for Earthquake Engineering Simulation (OpenSees), [Internet]. 2013.Available from: http://opensees.berkeley.edu/.
8.NFPA-13. Standard for the installation of Sprinkler System, National Fire Protection Association, MA, 2007 Edition. c2007.
9.Parulekar YM and et al. Seismic Response Evaluation of Safety Related Nuclear Structure with Yielding Dampers using Linearization Techniques. 20th International Conference on Structural Mechanics in Reactor Technology (SMiRT 20). Espoo, Finland, August 9-14. c2009.
10.PEER-NRG NGA. Pacific Earthquake Engineering Research Center: NGA Database. [Internet]. 2009.Available from: http://peer.berkeley.edu/nga/.
11.Wood RL, Hutchinson TC, Hoehler MS, Cyclic Load and Crack Protocols for Anchored Nonstructural Components and Systems. Structural Systems Research Project Report Series, SSRP 09/12. Department of Structural Engineering, University of California, San Diego, La Jolla, California. c2009.
12.Wasilewski RJ. Seismic Restraints for piping System. ASHRAE Transactions Part 1B. 1998;104:1273-1285.
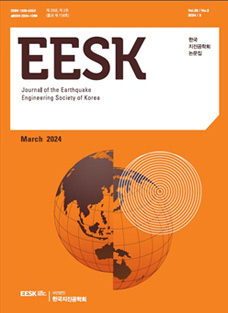
Frequency Bimonthly
Doi Prefix 10.5000/EESK
Year of Launching 1997
Publisher Earthquake Engineering Society of Korea



Online Submission
submission.eesk-j.or.kr
EESK
Earthquake Engineering Society of Korea